Antitop Quark
Composition :
Statistics : Generation :
Family :
Interaction forces :
Symbol :
Antiparticle :
Mass :
Decays into :
Electric charge :
Color charge
Spin :
Weak isospin :
Elementary antiparticle
Fermionic
Thrid
Quark
strong, weak,
electromagnetic force
gravity
t
Top quark ( t )
172,76 ± 0,3 GeV/c²
bottom quark (99,8%)
strabge quark (0,17 %)
down quark (0,007%)
-²∕₃ e
Yes
¹/₂
LH = +¹/₂; RH = 0
_
General
Mass and Energy:
Similar to the top quark, the antitop quark is extremely massive, with a mass close to 173 GeV/c². Its mass-energy equivalence, as described by Einstein's E=mc², means that it possesses a significant amount of energy.
Electric Charge:
Unlike the top quark, which carries an electric charge of +2/3e, the antitop quark has an electric charge of -2/3e. This negative electric charge complements the positive charge of the top quark.
Flavor and Color Charge:
The antitop quark is part of the "third generation" of quarks, just like the top quark. In the framework of quantum chromodynamics (QCD), quarks carry a "color" charge, which represents their participation in the strong nuclear force.
Strong Force Interactions:
Antitop quarks, like top quarks, interact via the strong nuclear force (QCD), mediated by gluons. These interactions are characterized by confinement, meaning quarks are never observed as free particles but are confined within color-neutral hadrons.
Weak Force Interactions:
The antitop quark also participates in weak force interactions, mediated by W and Z bosons. These interactions involve processes like beta decay and are responsible for various particle transformations.
Quantum Uncertainty:
Just as with top quarks, antitop quarks are subject to Heisenberg's uncertainty principle. This principle states that precise knowledge of both position and momentum of a particle is not possible, with the uncertainty in energy and time being particularly important for particles like antitop quarks with high energy and short lifetimes.
Short Lifetime:
Antitop quarks, like top quarks, are extremely short-lived, with a lifetime of about 5 x 10^(-25) seconds. This short lifetime results in rapid decay via the weak force, preventing their combination with other quarks to form hadrons.
Combinations with antitop quarks
Antitop quarks (t-bar) can combine with other particles to form various composite systems, such as hadrons or other particles. Let's provide a detailed overview of possible particle combinations composed of at least one antitop quark:
Top-Antitop Quark Pair (t-tbar):
The combination of a top quark and an antitop quark forms a top-antitop quark pair. These pairs are often created in high-energy particle collisions, such as those in particle accelerators. They are essential in the study of top quark properties and the validation of the Standard Model.
Antitop Mesons (T-Mesons):
A top meson is a composite particle composed of a top quark and an antiquark, which can be any quark of the appropriate charge. The most common antitop mesons are:
Top mesons are color-neutral and can be created in high-energy collisions. However, their short lifetimes make them challenging to observe directly.
Hadronic Decays of Top Quarks:
When top quarks decay, they produce a W boson and a bottom quark. This bottom quark can combine with an antibottom quark (bbar) to form a B meson. Therefore, you can have particles like B+ mesons and B0 mesons originating from the decay of a top quark.
Tetraquark States:
In theory, it's possible to have tetraquark states, which are exotic hadrons composed of four quarks, including an antitop quark. Tetraquark states have not been definitively observed yet, but they are part of ongoing research in particle physics.
Pentaquark States:
Pentaquark states are even more exotic, composed of five quarks, including an antitop quark. Like tetraquarks, the existence of pentaquarks is still a topic of research and investigation.
Resonances and Other High-Energy States:
In high-energy particle collisions, such as those at the Large Hadron Collider (LHC), various resonance states can be created as antitop quarks interact with other particles. These states are typically short-lived and decay into lighter particles.
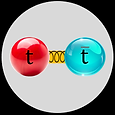
Figure 168 - Top-Antitop Quark Pair
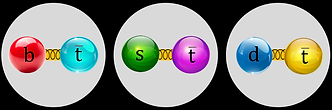
Figure 169 - Antitiop Mesons
Creation of antitop quarks
Top Quark Pair Production (t-tbar):
The most common process for creating antitop quarks is through top quark-antitop quark pair production. This occurs in high-energy proton-proton or proton-antiproton collisions at particle accelerators like the Large Hadron Collider (LHC) or the Tevatron (which has since been decommissioned).
In these collisions, the tremendous energy available can lead to the creation of a top quark and an antitop quark pair. This process is described by quantum chromodynamics (QCD) and is mediated by the exchange of gluons, which carry the strong nuclear force.
Single Antitop Quark Production:
Antitop quarks can also be produced singly, without a corresponding top quark. This process can occur in association with a W boson, and it is known as "s-channel" or "t-channel" single antitop quark production. It involves the interaction between a quark and an antiquark, resulting in an antitop quark and other particles.
Associated Production with Other Particles:
Antitop quarks can be produced in association with other particles, such as a W boson or a Higgs boson. These associated production processes are important in understanding the interactions between top quarks, antitop quarks, and other particles.
Heavy Flavor Production and Decays:
In some processes, heavy-flavor (bottom or charm) quarks can be produced in association with antitop quarks. These heavy quarks may subsequently decay, producing antitop quarks as part of their decay products.
Higher-Order Processes and Loop Diagrams:
At very high energies and in highly complex interactions, antitop quarks can be created through higher-order processes and loop diagrams involving the exchange of virtual particles. These processes contribute to the understanding of particle physics at the quantum level.
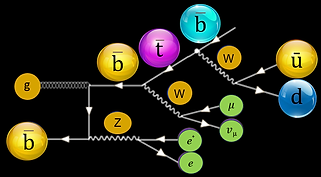
Figure 171 - t-channel single antitop quark production
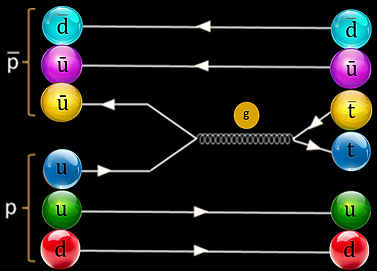
Figure 170 - Top Quark pair production via proton and antiproton

Figure 172 - Associated antitop production
Decay of antitop quarks
The antitop quark (t-bar) can decay into various particles through different decay modes, primarily mediated by the weak force, which is responsible for the transformation of quarks into other particles. Here, I'll describe some of the most common decay modes of the antitop quark and the resulting particles:
Leptonic Decay:
In a leptonic decay of the antitop quark, the primary decay products involve a charged lepton (e.g., an electron or muon) and a corresponding neutrino. The steps involved in this decay are as follows:
a. The antitop quark (t-bar) decays into a W⁻ boson.
b. The W⁻ boson subsequently decays into a charged lepton (e.g., an electron or muon) and its corresponding neutrino. For instance:
W⁻ → e⁻ + νₑ (electron neutrino)
W⁻ → μ⁻ + νₘ (muon neutrino)
In this scenario, the final-state particles are the charged lepton and the neutrino.
Hadronic Decay:
In a hadronic decay of the antitop quark, the primary decay products consist of quarks. This can result in various combinations of quarks. The steps are as follows:
a. The antitop quark (t-bar) decays into a W⁻ boson.
b. The W⁻ boson then decays into quarks. It can produce different combinations of quarks, which may include down-type (e.g., down, strange, bottom) and up-type (e.g., up, charm, top) quarks. For example:
W⁻ → b + u
W⁻ → s + c
The final state in hadronic decays typically includes jets of hadrons (e.g., pions, protons, and kaons) formed by the hadronization of the quarks.
Mixed Decay:
In some cases, the antitop quark can undergo a mixed decay, resulting in a combination of leptons and quarks. For example, one of the W⁻ boson decays into a charged lepton and its corresponding neutrino, while the other W⁻ boson decays into quarks.
The t-bar decays into a W⁻ boson and a bottom quark.
The W⁻ boson decays into a charged lepton (e.g., an electron or muon) and its corresponding neutrino. c. The W⁻ boson also decays into quarks, typically forming hadronic jets.
In all of these decay modes, the antitop quark transforms into other particles, obeying the principles of the weak force and conservation of charge, energy, and momentum. The specific particles in the final state can vary from one decay to another, and the probabilities of different decay modes depend on the properties of the antitop quark, such as its mass and electric charge.
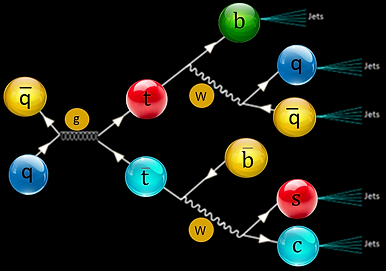
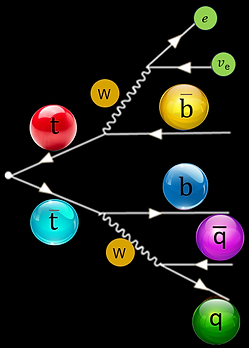
Figure 173 - Antitop leptonic decay
Figure 174 - Hadronic decay of the antitop quark
Annihilation of antitop quarks
Annihilation of an antitop quark (t-bar) is a theoretical concept that is not commonly observed in particle physics experiments. Annihilation typically refers to the process in which a particle and its corresponding antiparticle collide and transform their rest mass energy into other particles or radiation. In the case of a top quark and an antitop quark, their annihilation is a rare process due to their extremely high mass and short lifetime. Instead, the top quark and antitop quark more commonly decay into other particles, as described in the previous response.
However, if we were to consider the theoretical concept of top-antitop quark annihilation, it would involve the following process:
Pair Production: In high-energy particle collisions, such as those occurring at particle accelerators like the Large Hadron Collider (LHC), top quarks and antitop quarks can be created in pairs due to strong interactions. These collisions provide the necessary energy to produce these massive quarks.
Annihilation Interaction: In the extremely rare event that a top quark and an antitop quark come close to each other, they can undergo an annihilation interaction. This interaction would typically involve the strong force, which is responsible for binding quarks within hadrons, and would require the exchange of gluons.
Annihilation Products: During the annihilation process, the top quark and antitop quark would transform their rest mass energy into other particles or radiation. These annihilation products could include various combinations of quarks, gluons, and other particles, depending on the specific interaction. The annihilation process would obey the principles of conservation of energy, momentum, and other quantum numbers.
Fusion of antitop quarks
The fusion of antitop quarks is not a process that occurs in the framework of the Standard Model of particle physics, and it is not a concept typically discussed in particle physics. Antitop quarks, like top quarks, are fundamental particles governed by the fundamental forces of the Standard Model, and they do not readily undergo fusion as, for example, atomic nuclei do in nuclear fusion.
In the Standard Model, quarks (including top and antitop quarks) interact through the strong nuclear force mediated by gluons. This interaction is described by quantum chromodynamics (QCD), which governs the behavior of quarks and gluons within hadrons (composite particles like protons and neutrons) and their interactions in particle collisions. Quarks within a hadron are confined, and they do not exist in isolation.
In particle collisions and interactions at high-energy particle accelerators, top and antitop quarks are typically created in pairs (top-antitop quark pairs) through strong interactions, and they do not fuse together like atomic nuclei in fusion processes. Instead, these quarks primarily decay via weak interactions, as described in previous responses, leading to the production of various particles.
While theoretical physics explores exotic scenarios beyond the Standard Model, such as the behavior of particles at extremely high energies or in extreme conditions, the fusion of antitop quarks is not a process currently considered within the scope of particle physics. Particle physicists focus on understanding and characterizing the known interactions and decays of fundamental particles within the framework of the Standard Model.