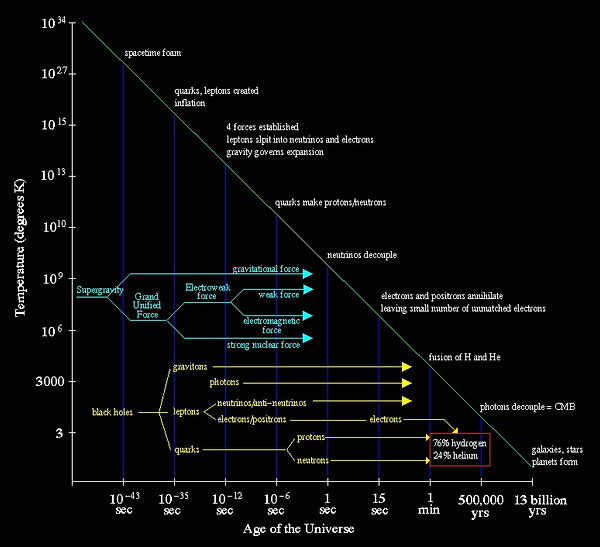
[4]
5 ELECTROWEAK EPOCH
5.1 General
During the electroweak epoch, the universe's temperature had decreased sufficiently (1.85 x 10¹⁵ K) for the strong force to split from the electroweak interaction. However, the temperature remained high enough for electromagnetism and the weak interaction to stay combined as a single electroweak interaction, above the critical temperature for electroweak symmetry breaking (159.5±1.5 GeV) according to the Standard Model of particle physics.
The exact timing of the electroweak epoch is debated among cosmologists. Some suggest it began at the onset of the inflationary epoch, around 10⁻³⁶ seconds following the Big Bang. Others argue it occurred closer to 10⁻³² seconds after the Big Bang, when the inflaton field's potential energy, responsible for the universe's inflation during the inflationary epoch, was released.
During this epoch, particle interactions were energetic enough to produce numerous exotic particles, such as W and Z bosons and Higgs bosons. As the universe expanded and cooled, these interactions became less energetic, and by the time the universe was approximately 10⁻¹² seconds old, the creation of W and Z bosons became undetectable. The remaining W and Z bosons decayed rapidly, and the weak interaction evolved into a short-range force in the subsequent quark epoch.
During the electroweak epoch, significant changes occurred in the fundamental forces of the universe. The high-energy environment allowed for unique interactions and transformations in these forces. Here's an overview of the changes that took place in the forces during the electroweak epoch:
5.1.1 Separation of the strong force
At the beginning of the electroweak epoch, the temperature of the universe had decreased enough for the strong force to separate from the electroweak interaction. The strong force, also known as the strong nuclear force, is responsible for binding quarks together to form protons and neutrons, and for holding atomic nuclei together.
5.1.2 Electroweak interaction
The electroweak interaction or electroweak force is the unified description of two of the four known fundamental interactions of nature: electromagnetism and the weak interaction. Although these two forces appear very different at everyday low energies, the theory models them as two different aspects of the same force. Above the unification energy, on the order of 246 GeV, they would merge into a single force. Thus, if the temperature is high enough – approximately 10¹⁵ K – then the electromagnetic force and weak force merge into a combined electroweak force. During the quark epoch (shortly after the Big Bang), the electroweak force split into the electromagnetic and weak force. It is thought that the required temperature of 10¹⁵ K has not been seen widely throughout the universe since before the quark epoch, and currently the highest human-made temperature in thermal equilibrium is around 5.5x1012 K (from the Large Hadron Collider).
Sheldon Glashow, Abdus Salam, and Steven Weinberg were awarded the 1979 Nobel Prize in Physics for their contributions to the unification of the weak and electromagnetic interaction between elementary particles, known as the Weinberg–Salam theory. The existence of the electroweak interactions was experimentally established in two stages, the first being the discovery of neutral currents in neutrino scattering by the Gargamelle collaboration in 1973, and the second in 1983 by the UA1 and the UA2 collaborations that involved the discovery of the W and Z gauge bosons in proton–antiproton collisions at the converted Super Proton Synchrotron. In 1999, Gerardus 't Hooft and Martinus Veltman were awarded the Nobel prize for showing that the electroweak theory is renormalizable.
5.1.3 Electroweak symmetry breaking
As the universe continued to expand and cool, the temperature eventually fell below the critical point for electroweak symmetry breaking. This caused the Higgs field to acquire a nonzero vacuum expectation value, leading to the breaking of electroweak symmetry. The once unified electroweak interaction then separated into two distinct forces: electromagnetism, mediated by photons, and the weak interaction, mediated by W and Z bosons.
5.1.4 Transformation of the weak interaction
After electroweak symmetry breaking, the remaining W and Z bosons decayed rapidly, causing the weak interaction to evolve into a short-range force. This short-range force would later play a role in processes such as beta decay, which involves the transformation of neutrons into protons or vice versa.
The electroweak epoch concluded with an electroweak phase transition of uncertain nature. If it was a first-order transition, it could generate a gravitational wave background. This phase transition might also be a source of baryogenesis, given that the Sakharov conditions are met.
In the minimal Standard Model, the electroweak epoch transition was neither a first- nor second-order phase transition but rather a continuous crossover. This scenario precludes baryogenesis or the production of an observable gravitational wave background. However, many extensions to the Standard Model, such as supersymmetry and the two-Higgs-doublet model, involve a first-order electroweak phase transition (though additional CP violation is necessary).
5.2 Electroweak epoch and matter
During the electroweak epoch, the universe's temperature was still high enough for various particle interactions to occur, leading to the creation of different particle species. Particle interactions during this period were energetic enough to produce a plethora of exotic particles, including W and Z bosons, Higgs bosons, and other elementary particles. Here's a detailed description of what happened with particle creation during the electroweak epoch:
-
Quark-gluon plasma: As the universe expanded and cooled down, the potential energy of the inflaton field was released, filling the universe with a dense, hot quark-gluon plasma. Quarks and gluons, the fundamental constituents of protons and neutrons, were free to move around and interact with each other at these high temperatures.
-
Electroweak interaction: During the electroweak epoch, the temperature of the universe was above the critical temperature for electroweak symmetry breaking. This meant that electromagnetism and the weak interaction had not yet separated and remained combined as a single force known as the electroweak interaction.
-
Creation of exotic particles: The high energy of particle interactions during this epoch allowed the production of various exotic particles. These included W and Z bosons, which are responsible for mediating the weak nuclear force, and Higgs bosons, which are responsible for providing other particles with mass through the Higgs mechanism. These particles were created in large numbers as a result of the energetic collisions between quarks, gluons, and other particles.
-
Decrease in particle creation: As the universe continued to expand and cool, particle interactions became less energetic. Around 10^-12 seconds after the Big Bang, the creation of W and Z bosons ceased to occur at observable rates due to the reduced energy of these interactions.
-
Decay of W and Z bosons: The remaining W and Z bosons present during the electroweak epoch decayed quickly into lighter particles. As a result, the weak interaction transformed into a short-range force that would later play a role in processes such as beta decay during the subsequent quark epoch.
Throughout the electroweak epoch, the universe underwent a series of transitions as the temperature decreased and the various forces began to differentiate. These transitions eventually led to the formation of the distinct forces and particle interactions that we observe today.
Literature :
-
"The First Three Minutes" by Steven Weinberg - This classic book provides a detailed overview of the first few minutes of the universe, including the electroweak epoch.
-
"The Standard Model and Beyond" by Paul Langacker - This book provides a comprehensive introduction to the standard model of particle physics, including the electroweak theory.
-
"The Quantum Universe: Everything That Can Happen Does Happen" by Tony Hey and Patrick Walters - This book provides a non-technical introduction to the electroweak theory and its implications for the universe.
-
"The Electroweak Interaction" by Walter Greiner, Berndt Müller - This book provides a comprehensive introduction to the electroweak theory and its implications for particle physics.
-
"Electroweak Interactions and Unified Theories" by Jean Iliopoulos - This book provides a detailed introduction to the electroweak theory and its implications for our understanding of the universe.
-
ectroweak Symmetry Breaking: The Bottom-Up Approach" by Marco Battaglia - This book provides a detailed introduction to the Higgs mechanism and the electroweak symmetry breaking in the context of the standard model.
-
"The Electroweak Interaction: An Introduction to the Physics of Quarks and Leptons" by Peter Renton - This book provides a comprehensive introduction to the electroweak interaction and its implications for our understanding of the universe.
-
"The Electroweak Theory" by Alessandro Bettini - This book provides a detailed introduction to the electroweak theory and its implications for particle physics, including the Higgs mechanism and the unification of the weak and electromagnetic interactions.
-
Electroweak symmetry breaking and Higgs physics. Michael Spira and Peter M. Zerwas (1997)

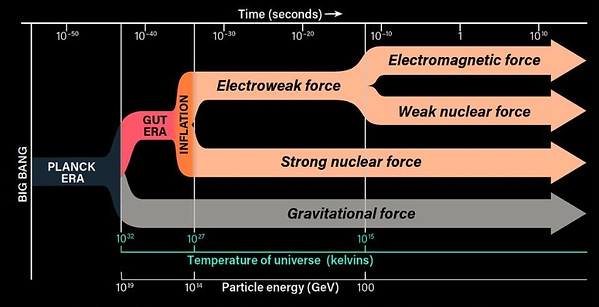
Literature
References
[1] Wikipedia
[2] L. D. McLerran, M. E. Shaposhnikov, N. Turok and M. B. Voloshin (1991). "Why the baryon asymmetry of the universe is approximately 10**-10". Phys. Lett. B. 256: 451–456. doi:10.1016/0370-2693(91)91794-V.
[3] Morrissey, David E. and Ramsey-Musolf, Michael J. (2012). "Electroweak baryogenesis". New J. Phys. 14 (12): 12500. arXiv:1206.2942. Bibcode:2012NJPh...14l5003M. doi:10.1088/1367-2630/14/12/125003. S2CID 119230032.
[4] Course HC209 21st Century Science. Univerity of Oregon. Prof. James Schombert
[5] The Anatomy of electro-weak symmetry breaking. I: The Higgs boson in the standard model. A. Djouadi (2005)