2 PLANCK EPOCH
2.1 General
The Planck epoch constitutes a phase in classical (non-inflationary) Big Bang cosmology, transpiring immediately following the genesis of the observed universe. This period, spanning from time zero to approximately 10⁻⁴³ s post-Big Bang, is characterised by exceedingly elevated temperatures and energy densities, reaching values on the order of the Planck temperature (approximately 1.42 x 10³² Kelvin) and the Planck density (approximately 5.16 x 10⁹⁶ kg/m³), respectively.
During the Planck epoch, the formation of customary subatomic particles was hindered, owing to the prevailing extreme conditions. Concurrently, the four fundamental forces — namely, gravitation, electromagnetism, weak nuclear force, and strong nuclear force — were conjectured to have been unified, thereby constituting a singular, primordial force. The comprehension of physics under these extraordinary temperatures remains largely inadequate, with multiple hypotheses proposing disparate scenarios.
The standard Big Bang cosmology anticipates a gravitational singularity preceding the Planck epoch, a notion predicated on the general theory of relativity. Nonetheless, this theory is presumed to falter during the Planck epoch due to quantum effects, necessitating the development of a consistent quantum gravity framework to accurately describe the dynamics at play during this time.
Inflationary cosmological models present alternative temporal progressions that deviate from the chronology delineated in traditional Big Bang cosmology, specifically for time intervals before the cessation of inflation (approximately 10⁻³² seconds post-Big Bang). Theoretical constructs aiming to elucidate the universe and its governing physics during the Planck epoch are predominantly speculative and reside within the realm of "New Physics." Noteworthy examples include the Hartle-Hawking initial state, the string theory landscape, string gas cosmology, and the ekpyrotic universe.
2.2 The Hartle-Hawking initial state
The Hartle-Hawking initial state, also known as the "no-boundary proposal," is a theoretical construct in quantum cosmology, proposed by James Hartle and Stephen Hawking in 1983. This model aims to provide a description of the universe's initial conditions, addressing the singularity problem associated with the Big Bang in the context of quantum mechanics.
In classical Big Bang cosmology, the universe is believed to have originated from a singular point with infinite density and temperature. However, classical theories, including general relativity, are inadequate for describing such singularities. The Hartle-Hawking initial state postulates that the universe did not have a definite beginning at a singular point. Instead, it suggests that time and space are interconnected and emerge smoothly from a single four-dimensional Euclidean space.
The Hartle-Hawking proposal utilizes a mathematical framework known as "imaginary time." By substituting real time with imaginary time (where imaginary time is obtained by multiplying real time by the imaginary unit i, the square root of -1), the model effectively eliminates the initial singularity problem. The universe is thus conceived as a compact, finite, and smoothly connected four-dimensional manifold without any boundaries or singularities.
According to the Hartle-Hawking initial state, the universe emerged in a highly ordered and uniform state, characterized by minimal entropy. As the universe evolved, it transitioned from a Euclidean space with imaginary time to a Lorentzian space with real time, and the classical notions of space and time became applicable. The model remains consistent with both general relativity and quantum mechanics, while avoiding the singularity issue.
Literature
The Hartle-Hawking initial state is a significant topic in quantum cosmology, and numerous scientific publications have contributed to its development and understanding. Here is a list of some key literature on the subject:
-
Hartle, J.B., and Hawking, S.W. (1983). "Wave Function of the Universe." Physical Review D, 28(12), 2960-2975.
-
Hawking, S.W. (1984). "The Quantum State of the Universe." Nuclear Physics B, 239(1), 257-276.
-
Vilenkin, A. (1984). "Quantum Creation of Universes." Physical Review D, 30(2), 509-511.
-
Halliwell, J.J., and Hawking, S.W. (1985). "Origin of Structure in the Universe." Physical Review D, 31(8), 1777-1791.
-
Hartle, J.B., Hawking, S.W., and Hertog, T. (2008). "Classical Universes of the No-Boundary Quantum State." Physical Review D, 77(12), 123537.
-
Hertog, T., and Hartle, J.B. (2012). "Holographic No-Boundary Measure." Journal of Cosmology and Astroparticle Physics, 2012(02), 026.
These articles provide a starting point for understanding the Hartle-Hawking initial state and its implications for quantum cosmology. The initial papers by Hartle and Hawking outline the foundational concepts, while subsequent works delve into various aspects, applications, and refinements of the theory.
2.3 The String Theory landscape
The string theory landscape refers to the vast collection of possible vacuum states and configurations allowed by string theory, a theoretical framework that unifies quantum mechanics and general relativity. String theory posits that the fundamental constituents of the universe are not point-like particles but rather one-dimensional, vibrating strings. These strings can oscillate in various modes, with each mode corresponding to a different particle or force.
In the context of the Planck epoch, the string theory landscape is relevant because it may provide insights into the initial conditions and the evolution of the universe during this early phase. String theory, which encompasses both quantum mechanics and gravity, offers a promising avenue for exploring the behaviour of the universe under these extreme conditions.
The landscape of string theory comprises an enormous number of possible vacuum states, each characterised by distinct values of physical parameters, such as the cosmological constant and particle masses. This multitude of vacua arises from the various ways in which extra dimensions, which are a crucial component of string theory, can be compactified or "curled up" at small scales. As a result, each vacuum state may yield a unique low-energy effective field theory, potentially leading to a diverse array of physical consequences.
One implication of the string theory landscape in the context of the Planck epoch is the concept of "eternal inflation." In this scenario, different regions of the universe could be transitioning between various vacuum states, resulting in a multiverse with distinct local laws of physics. The universe we observe may then be just one of many "pocket universes" within this larger multiverse, each with its unique vacuum state and physical parameters.
Despite its potential to address fundamental questions about the universe's origins, the string theory landscape remains speculative and a subject of ongoing research. Several challenges, such as the lack of experimental verification and the difficulty in making concrete predictions, still persist. Nevertheless, the string theory landscape offers a fascinating avenue for exploring the early universe's evolution and the extreme conditions that prevailed during the Planck epoch.
Literature :
-
Susskind, L. (2003). "The Anthropic Landscape of String Theory." In The Davis Meeting On Cosmic Inflation.
-
Bousso, R., and Polchinski, J. (2000). "Quantization of Four-Form Fluxes and Dynamical Neutralization of the Cosmological Constant." Journal of High Energy Physics, 2000(6), 006.
-
Denef, F., and Douglas, M. R. (2004). "Distributions of Flux Vacua." Journal of High Energy Physics, 2004(5), 072.
-
Douglas, M. R., and Kachru, S. (2007). "Flux Compactification." Reviews of Modern Physics, 79(1), 733-796. URL: https://arxiv.org/abs/hep-th/0610102
-
Grana, M. (2005). "Flux Compactifications in String Theory: A Comprehensive Review." Physics Reports, 423(2), 91-158.
-
Kallosh, R., and Linde, A. (2004). "Landscape, the Scale of SUSY Breaking, and Inflation." Journal of High Energy Physics, 2004(12), 004.
2.4 The String Gas Cosmology
String gas cosmology is a theoretical framework that combines elements of string theory and cosmology to investigate the early universe's evolution, particularly during the Planck epoch. This approach was initially proposed by Robert H. Brandenberger and Cumrun Vafa in 1989 as an alternative to inflationary models in explaining the observed large-scale structure of the universe.
In the context of the Planck epoch, string gas cosmology posits that the universe began in a high-energy state where the fundamental constituents were one-dimensional, vibrating strings. These strings oscillate in various modes, each corresponding to a distinct particle or force. During the Planck epoch, when the four fundamental forces are believed to have been unified, string gas cosmology provides a means to explore the behaviour of the universe under extreme conditions, where our current understanding of physics is limited.
The key ingredient of string gas cosmology is the presence of a "string gas" – a hot, dense, and highly excited state of strings that fill the universe. In the Planck epoch, these strings are in thermal equilibrium, with the energy being equally distributed among various oscillation modes. As the universe expands and cools, the string gas undergoes a phase transition, where the energy of the strings is transferred to a lower-dimensional set of oscillation modes. This phase transition leads to the formation of stable and long-lived strings, which later evolve into cosmic structures.
One of the main goals of string gas cosmology is to provide a consistent explanation for generating primordial fluctuations that seeded the formation of cosmic structures, such as galaxies and galaxy clusters. In this framework, the fluctuations arise from the statistical properties of the string gas, as opposed to the quantum fluctuations in the inflationary paradigm. Aditionally, string gas cosmology can provide insights into the stabilisation and size of the extra dimensions required by string theory.
Literature
-
Brandenberger, R., and Vafa, C. (1989). "Superstrings in the Early Universe." Nuclear Physics B, 316(2), 391-410.
-
Brandenberger, R. (2008). "String Gas Cosmology." Progress of Theoretical Physics, Vol. 111, No. 4, April 2004
-
Battefeld, T., and Watson, S. (2009). "String Gas Cosmology." Reviews of Modern Physics, 78(3), 435-454.
-
Brandenberger, R. (2011). "String Gas Cosmology after Planck."
-
Brandenberger, R. (2012). "The Matter Bounce Alternative to Inflationary Cosmology." Physics of the Dark Universe, 1(1), 3-11.
-
Brandenberger, R., and Peter, P. (2016). "Bouncing Cosmologies: Progress and Problems." Foundations of Physics, 47(6), 797-850.
-
Brandenberger R. (2009) "Alternatives to cosmological inflation". (2009)
2.5 The Ekpyrotic universe
The term "ekpyrosis" originates from the ancient Greek word meaning "conflagration," signifying a fiery event. The ekpyrotic scenario was first proposed by Paul Steinhardt, Neil Turok, Justin Khoury, and Burt Ovrut in 2001, based on the principles of string and M-theory.
In the context of the Planck epoch, the ekpyrotic universe provides an alternative framework to explore the extreme conditions that prevailed when the four fundamental forces were presumably unified. According to the ekpyrotic model, the universe we observe is a three-dimensional "brane" (short for "membrane") embedded within a higher-dimensional space called the "bulk" or "hyperspace." Our universe is one of the two parallel branes separated by a small distance in the extra dimension. The model posits that the Big Bang event was a consequence of a collision between these two branes.
During the Planck epoch, the two branes approach each other, drawn together by a force mediated through the extra dimensions. As the branes collide, their kinetic energy is converted into matter and radiation, resulting in a hot, dense state similar to the initial conditions in the standard Big Bang model. This collision effectively replaces the singularity problem associated with the conventional Big Bang, providing an alternative explanation for the universe's origins.
The ekpyrotic model can potentially address several issues that the inflationary paradigm seeks to resolve, such as the flatness, horizon, and monopole problems. It also provides a mechanism for generating primordial density perturbations that lead to the formation of cosmic structures. In the ekpyrotic scenario, these perturbations arise from the quantum fluctuations of the branes' relative position in the extra dimensions, rather than the inflationary quantum fluctuations.
Literature :
-
Lehners, J.-L. (2008). "Ekpyrotic and Cyclic Cosmology." Physics Reports, 465(1-3), 223-263.
-
Buchbinder, E. I., Khoury, J., and Ovrut, B. A. (2007). "New Ekpyrotic Cosmology." Physical Review D, 76(12), 123503.
-
Battarra, L., Koehn, M., Lehners, J.-L., and Ovrut, B. A. (2014). "Cosmological Perturbations Through a Non-Singular Ghost-Condensate/Galileon Bounce." Journal of Cosmology and Astroparticle Physics, 2014(07), 007.
-
Ijjas, A., and Steinhardt, P. J. (2015). "The Anamorphic Universe."
-
Fertig, A., Lehners, J.-L., Mallwitz, E., and Wilson-Ewing, E. (2016). "Converting Entropy to Curvature Perturbations after a Cosmic Bounce." Journal of Cosmology and Astroparticle Physics, 2016(10), 005.
-
Brandenberger, R., and Peter, P. (2017). "Bouncing Cosmologies: Progress and Problems." Foundations of Physics, 47(6), 797-850.
-
Lehners, J.-L., and Wilson-Ewing, E. (2015). "Running of the Scalar Spectral Index in Bouncing Cosmologies"
-
Cai, Y.-F., and Wilson-Ewing, E. (2014). "A ΛCDM Bounce Scenario."

[2]
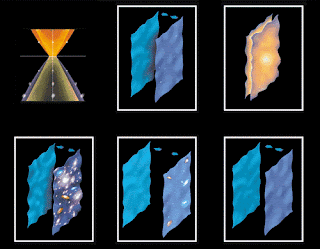
[3]
[1]
2.6 Planck epoch and matter
Our understanding of matter during the Planck epoch is limited due to the extreme conditions and the limitations of our current physical models. Further advances in theoretical physics, including the development of a quantum theory of gravity, may help shed light on the nature of matter and the behavior of the universe during the Planck epoch.
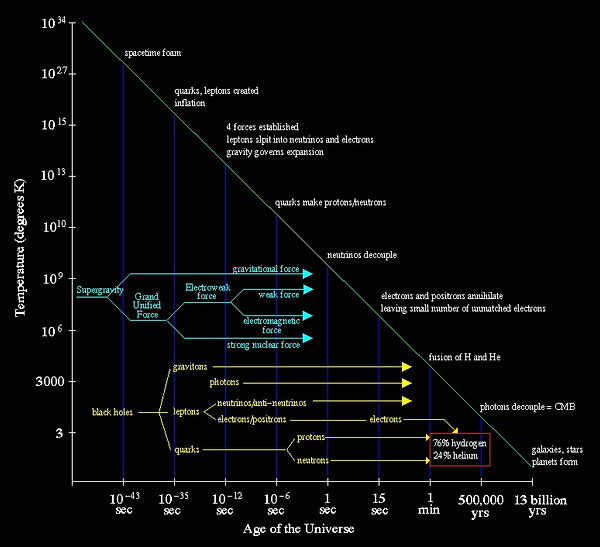
References
[1] Wikipedia
[2] Eventent one0teen. Cosmogony. YouTube URL : https://www.youtube.com/watch?v=SccjUqVyQYM
[3] The ekyprotic universe. Universal Encyclopedia (2018)
[4] The Planck era: Imagining our infant universe. Sten Odenwald. Astronomy April 2021