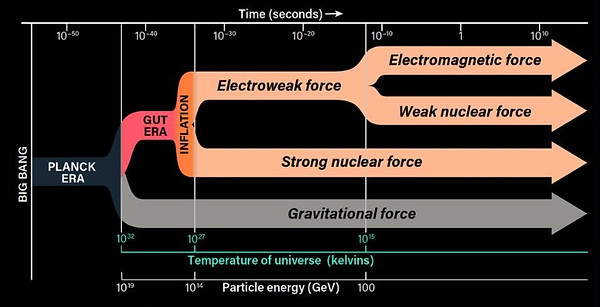
6 QUARK EPOCH [1]
6.1 General
The Quark epoch was the period in the evolution of the early universe when the fundamental interactions of gravitation, electromagnetism, the strong interaction and the weak interaction had taken their present forms, but the temperature of the universe was still too high (between around 10¹⁵ Kelvin at the beginningof the poch) to allow quarks to bind together to form hadrons.[2]
The quark epoch began approximately 10⁻¹² seconds after the Big Bang, when the preceding electroweak epoch ended as the electroweak interaction separated into the weak interaction and electromagnetism. The universe had a higher degree of stability.
During the quark epoch, the universe was filled with a dense, hot quark–gluon plasma, containing quarks, gluons, leptons and their antiparticles. Collisions between particles were too energetic to allow quarks to combine into mesons or baryons.
During the quark epoch, baryogenesis, the creation of matter-antimatter asymmetry, is thought to have occurred. This process is still not fully understood, but it is believed to have involved a violation of the conservation of baryon number, which is the number of protons and neutrons in the universe. This violation would have resulted in a small excess of matter over antimatter, which allowed the matter in the universe to survive while most of the antimatter annihilated with matter, leaving a small amount of matter behind.
6.2 Baryogenesis
The creation of matter-antimatter asymmetry, is believed to have occurred during the quark epoch, but it is also thought tohave occurred during other periods in the history of the universe. The exact mechanism of baryogenesis is not fully understood, but it is believed to involve a violation of the conservation of baryon number, which is the number of protons and neutrons in the universe.
The central question to Baryogenesis is what causes the preference for matter over antimatter in the universe, as well as the magnitude of this asymmetry. An important quantifier is the asymmetry parameter, given by
where and refer to the number density of baryons and antibaryons respectively and is the number density of cosmic background radiation photons. [3]
According to the Big Bang model, matter decoupled from the cosmic background radiation (CBR) at a temperature of roughly 3000 kelvin, corresponding to an average kinetic energy of 3000 K / (10.08×10³ K/eV) = 0.3 eV. After the decoupling, the total number of CBR photons remains constant. Therefore, due to space-time expansion, the photon density decreases. The photon density at equilibrium temperature T per cubic centimeter, is given by
with as the Boltzmann constant, ħ as the Planck constant divided by 2π and c as the speed of light in vacuum, and ζ(3) as Apéry's constant.[3] At the current CBR photon temperature of 2.725 K, this corresponds to a photon density nγ of around 411 CBR photons per cubic centimeter.
Therefore, the asymmetry parameter η, as defined above, is not the "best" parameter. Instead, the preferred asymmetry parameter uses the entropy density s,
because the entropy density of the universe remained reasonably constant throughout most of its evolution. The entropy density is
with p and ρ as the pressure and density from the energy density tensor Tμν, and g⁎ as the effective number of degrees of freedom for "massless" particles at temperature T (in so far as mc2 ≪ kBT holds),
for bosons and fermions with gi and gj degrees of freedom at temperatures Ti and Tj respectively. At the present epoch, s = 7.04 nγ
One hypothesis for baryogenesis is that it occurred during a phase transition in the early universe. A phase transition is a change in the state of matter, such as the transition from a liquid to a gas. During a phase transition, the conditions of the universe change rapidly, and this change could have led to the creation of matter-antimatter asymmetry.
Another hypothesis is that baryogenesis occurred during the electroweak epoch, which was the period following the quark epoch when the electroweak force separated into the electromagnetic force and the weak force. During this epoch, the conditions of the universe were still hot and dense, and there were many interactions between particles that could have led to the creation of matter-antimatter asymmetry.
Overall, while the quark epoch is considered a likely period for baryogenesis, it is thought to have occurred during other periods in the history of the universe as well. Further research and investigation are needed to fully understand the process of baryogenesis and its occurrence throughout the history of the universe.
The quark epoch ended when the universe was about 10−6 seconds old, when the average energy of particle interactions had fallen below the binding energy of hadrons. The following period, when quarks became confined within hadrons, is known as the hadron epoch.
6.3 The Quark epoch and matter
-
Quark-Gluon Plasma: At the start of the quark epoch, the universe was in a state of extreme energy density and temperature. Under these conditions, quarks and gluons existed in a plasma state known as quark-gluon plasma, where they were not confined to form hadrons. The exact mechanism by which quarks and gluons were created during this period is still not fully understood, but it is believed that they were produced through processes such as cosmic inflation or particle-antiparticle annihilation reactions.
-
Confinement: As the universe cooled and expanded, the strong force separated from the electroweak force, causing quarks to become confined within hadrons such as protons and neutrons. This confinement marked the end of the quark epoch and the beginning of the hadron epoch.









6.4 Advised Literature
-
Gravitational mechanism for baryogenesis in the cosmological QCD phase transition. V. Antunes, I. Bediaga and M. Novello. (2016)
-
Describing the dynamics of the quark-gluon plasma using relativistic viscous hydrodynamics. Mohammad N. Yaseen (Kent State University) (2016)
-
Minimal cosmological masses for photons and gluons. Lorenzo Gallerani Resca (2019)
-
Traveling Through the Universe: Back in Time to the Quark-Gluon Plasma Era. Johann Rafelski and Jeremey Birrell
-
Dark Photon Production in the Quark Epoch. Nivedita Ghosh, M. C. Kumar, and R. K. Singh (2023)
-
Nucleation of Quark-Hadron Phase Transition in the Early Universe. Chia-Chen Chang and Li-Ming Cao (2022)
-
Quark-Hadron Transition in the Early Universe: Chemical Equilibrium and Beyond. Aritra Bandyopadhyay, Santabrata Pal, and Sudip Bhattacharyya (2021)
-
The Quark-Hadron Transition in a Strongly-Coupled Plasma from Gravity Duals. Aritra Kundu (2021)
-
Dark Photon Production in the Quark Epoch. Nivedita Ghosh, M. C. Kumar, and R. K. Singh (2023)
-
Nucleation of Quark-Hadron Phase Transition in the Early Universe. Chia-Chen Chang and Li-Ming Cao (2022)
-
Quark-Hadron Transition in the Early Universe: Chemical Equilibrium and Beyond. Aritra Bandyopadhyay, Santabrata Pal, and Sudip Bhattacharyya (2021)
-
The Quark-Hadron Transition in a Strongly-Coupled Plasma from Gravity Duals. Aritra Kundu (2021)
-
"The Early Universe" by Edward W. Kolb and Michael S. Turner, published in 1994 in the book "The Early Universe." This paper provides an overview of the early universe, including the quark epoch.
-
"The Quark-Hadron Phase Transition in the Early Universe" by Frithjof Karsch, published in 2002 in the journal "Lectures on QCD: Applications." This paper discusses the transition from the quark epoch to the hadron epoch, and the role of the quark-hadron phase transition.
-
"The Quark Epoch and the Origin of Structure" by David N. Spergel and Paul J. Steinhardt, published in 2000 in the journal "Physical Review Letters." This paper discusses the potential implications of the quark epoch for the formation of structure in the universe.
-
"The Early Universe: Facts and Fiction" by Andrei Linde, published in 2003 in the journal "Reviews of Modern Physics." This paper provides a broad overview of the early universe, including the quark epoch.
References
[1] Wikipedia
[2] Rafelski, Johann (October–November 2013). "Connecting QGP-Heavy Ion Physics to the Early Universe". Nuclear Physics B: Proceedings Supplements. 243–244: 155–162. arXiv:1306.2471. Bibcode:2013NuPhS.243..155R. doi:10.1016/j.nuclphysbps.2013.09.017. S2CID 118460277 – via ResearchGate.
[3] Cline, James M. (2006-11-22). "Baryogenesis". arXiv:hep-ph/0609145